The Maltese Government's launch of a draft "National Policy for the Deployment of Offshore Renewable Energy" for public consultation last year marks an important part of the process towards reaching carbon neutrality in Malta within the next 25 years or so. Offshore wind power generation would appear to be a key contributor in a future renewable technology mix for deployment off Maltese shorelines; more specifically in Malta's Exclusive Economic Zone (EEZ).
This article presents some insights on the nationally-financed R&I project "Hydro-pneumatic Energy Storage for Offshore Green Hydrogen Generation (HydroGenEration)", which is a two-year project concluding in mid-2024 and is financed by the Energy and Water Agency under the National Strategy for Research and Innovation in Energy and Water (2021-2030).
This project is underway at the University of Malta's Institute for Sustainable Energy in collaboration with the Department of Mechanical Engineering and the University's homegrown spinoff company FLASC B.V., based in the Netherlands.

We have heard about offshore fixed-foundation wind turbines and more recently, of the floating offshore type; a concept which has moved off the drawing board and into practice; an example being Equinor's Hywind Tampen wind park. Floating wind turbines open new horizons in terms of installation locations, given that deeper seas farther away from coastal zones can be utilised, thereby reducing the socio-environmental impacts and concerns that are associated with large wind turbines.
This is a game changer, especially for a country like Malta which has a high urban concentration along its coastlines.
The project "Hydro-pneumatic Energy Storage for Offshore Green Hydrogen Generation (HydroGenEration)" is a desk-based project focusing on floating wind power and green hydrogen as a zero-impact fuel produced in that same environment which supplies the primary energy source itself, i.e., water.
The terminology "Green Hydrogen" is used when the gas is produced from clean, carbon-free energy sources, such as wind or solar power. HydroGenEration, or HGE in short, is looking at floating offshore wind power as a means of supplying electrical power to an offshore hydrogen production and hydrogen storage facility for central Mediterranean waters, while still being close enough to the hydrogen fuel end-users.
The end-users are envisaged to be the maritime sector operators comprising motorised seafaring vessels of all types and size which, in the future, could potentially also transition from fossil fuel powered propulsion systems to hydrogen-driven motive power, thus decarbonising the maritime sector.
So, what is the novelty of the HydroGenEration concept system?
The novelty lies in stabilising the green electrical power being generated by the offshore renewables. It is well known that wind is an intermittent resource, and that its electrical output is unpredictable and highly variable. On the other hand, electrolyser technology, or the equipment which splits the water into hydrogen and oxygen, would operate more comfortably and enjoy a longer lifetime if powered by a steadier electrical supply and would benefit from operating with less shut-downs and start-ups.
Energy storage can contribute by balancing the excess renewable electricity produced by wind power and storing it, to release it at a time when there are shortfalls in the supply, i.e., when there is a higher demand for electricity than what can be provided by wind power at a certain instant. In other words, smoothening the intermittent renewable energy electricity to a more constant supply and reducing energy curtailment, or wastage, when there is energy in excess of users', or process, requirements.
The University of Malta has developed and patented a Hydro-Pneumatic Energy Storage (HPES) solution, known as the Floating Liquid Piston Accumulator using Seawater Under Compression (FLASC) technology, for storing and recovering electrical energy using pressurised seawater and compressed air.
The FLASC HPES system consists of an Energy Conversion Unit (ECU) with a pump/turbine and a Pressure Containment System (PCS) which stores the electrical energy in a pressurised gas/liquid medium. This energy storage system, which is specifically tailored for offshore deployment, can stabilise a fluctuating electrical energy supply such as that coming from renewables.
The HydroGenEration project is building on experience acquired in the earlier Malta Marittima-funded "Wind-driven Offshore Hydrogen Production with Electricity and Flow Stabilisation (WIND4H2)[1]" project, which had investigated the performance of an offshore green hydrogen production plant with HPES operating under North Sea wind conditions. The current investigation is now focusing more closely on modelling the HGE concept system's behaviour under Central Mediterranean climatic conditions, which are characterised by lower wind availability and higher variability.
Starting off with modelling the HydroGenEration concept system under North Sea conditions based on wind data from different offshore meteorological stations, the HGE project then moved on to model 10 MW offshore wind turbine performance under Mediterranean winds using 12 months of wind data captured by a Light Detection and Ranging (LiDAR) wind measurement system [2] located at Qalet Marku [3] on Malta's northern coastline for a height equivalent to 111 metres above the sea surface.
The Hydrogen Production Unit (HPU) comprises a Reverse Osmosis module to filter and desalinate the seawater, a Proton Exchange Membrane (PEM) electrolyser to separate the desalinated water into hydrogen and oxygen, and an Ionic Compressor to compress the hydrogen to a pressure of 700 bar for subsequent storage subsea on the seabed and eventual utilisation as a fuel for motive power. The HPU is envisaged to be co-located on the wind turbines' floaters, while in the case of the hydrogen storage, this was designed for installation on the seabed, which would offer a more secure option and better space utilisation on a number of counts. Figure 1 shows a schematic of the key components of one of the HydroGenEration concept system's clusters, with their respective inputs and outputs.
The numerical modelling involved supplying the hydrogen production unit (HPU) with the same electrical supply, but in two forms:
1. as a fluctuating wind energy supply coming directly from three 10 MW wind turbines with a rotor hub height of 119 metres above the sea surface and
2. from the same green energy source, but first fed through the FLASC HPES system, consisting of a hydro-pneumatic accumulator placed on the seabed and a topside Energy Conversion Unit (ECU) consisting in a pump/turbine placed on the floating wind turbine's floater.
These two electrical supply baselines enabled an assessment of the advantages, if any, of interfacing the FLASC HPES system into the offshore green hydrogen production process chain.

Schematic of the HydroGenEration project concept cluster showing the key modelling blocks with inputs and outputs of the various processes.
System sizing and optimisation followed; initially for a three 10 MW wind turbine cluster. Different electrolyser power ratings were modelled in combination with different FLASC HPES system scheduling windows, or 'hold times', and FLASC HPES system energy ratings. The initial results showed that using the FLASC energy storage system significantly reduced the number of electrolyser shutdowns and start-ups when compared to a direct feed of wind energy supplies, as shown in chart below. Moreover, the improved electrolyser utilisation rates were even more evident in a Mediterranean wind climate.

Comparison of the direct (fluctuating) power output from 3 x 10 MW wind turbine (in BLUE) cluster compared to the smoothened power output after storage and release by the FLASC HPES system (in RED) for Mediterranean wind conditions.
So, the integration of the FLASC HPES not only reduces the number of electrolyser stops and starts, but also cuts down on the time required for the electrolyser to ramp up after a start-up to reach normal hydrogen production rates once again, while also marginally improving hydrogen production rates and reducing the amount of electrical energy curtailed. Although minor, a 0.3% increase in the amount of hydrogen produced during the 12 months under evaluation was calculated. This would work out to some 5,300 kg more of hydrogen being produced by a single cluster, based on the 2016 wind climate. It is envisaged that electrolyser lifetime, and thus replacement costs, will also be improved by integrating HPES into the concept system's operational strategy.
Upscaling of such a modular three-turbine concept cluster could result in a linear or rectangular array of 10 floating wind turbines (i.e. three clusters) interconnected electrically in circular fashion for operational reliability. One redundant turbine will be included to supply ancillaries with power and also as a backup machine.
Modular FLASC HPES systems and multiple HPUs will be connected to single or multiple dispersed subsea hydrogen storage systems placed on the seabed. One or more fuelling buoys would allow for refuelling of seafaring vessels or alternatively hydrogen carriers which will take the hydrogen fuel produced by the conceptualised HGE system for transportation to end-users nearby, or outside the immediate vicinity of the offshore HGE plant itself. It is worth noting that wind farms and seafaring vessels require adequate buffer zones for the safe navigation and manoeuvring of such vessels.
If the hydrogen produced by the HydroGenEration project concept in the sample year were to be used in hydrogen fuel cell-driven cars, this would enable a distance of 557,718 thousand kilometers to be covered. This would correspond to the fuelling of about 102 thousand hydrogen powered cars, with a corresponding CO2 emissions reduction of 64,193 tons per year. This amounts to more than one third of the total emissions in this transport category.
The HGE project also involved a desk-based site selection exercise in order to identify potential locations that would offer the right combination of site conditions based on the Continental Shelf Department's definition of the extents and specific areas for offshore renewable energy project developments within Malta's Exclusive Economic Zone (EEZ).
Factors such as exposure to the local prevailing winds, bathymetry and indicative seabed composition, distance to ports and harbours, avoidance of subsea cables and pipelines, interactions with seaborne and aviation traffic and interactions with aquaculture and fisheries, were some of the factors used to map out a decision matrix to shortlist those locations which would appear to be more promising for the HydroGenEration concept system. Environmental factors, such as marine Special Areas of Conservation (SACs) and Special Protection Area (SPAs), etc., were also built into the decision-making process. Last but not least, the exercise kept in mind the need to supply the hydrogen fuel to end-users, so ship berthing and bunkering areas were also mapped out. A series of online Webinars were held with stakeholders over a three-day time frame to present the preliminary sites listed (see Figure 3) and to get feedback in terms of conflicts which might have been missed.
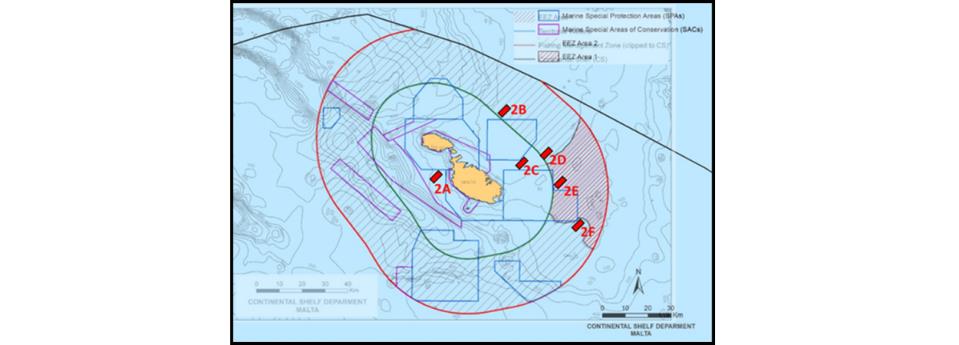
Site identification process for the HydroGenEration project concept showing the EEZ and shortlisted sites for a 10 x 10 MW wind turbine rectangular array (Basemap images courtesy of the "Preliminary Market Consultation (PMC) for the proposal of economic activities within Malta's Exclusive Economic Zone", Continental Shelf Department (PMC No. CSD/4/2022), Malta, 2 May 2022).
Project HydroGenEration is now in its final stages, during which an economic analysis will strive to determine the Levelised Cost of Hydrogen (LCOH), or the cost per kg of hydrogen produced (€/kg) by the HGE concept system operating in a local scenario.
The HGE team members are Dr Oleksii Pirotti, Ms Diane Scicluna, Dr Ing. Robert N. Farrugia, Prof. Tonio Sant and Dr Daniel Buhagiar.
The HGE project team also acknowledges the support of the University of Malta’s Project Support Office, the Corporate Research and Knowledge Transfer Office and of the Marketing, Communications and Alumni Office.
Project "Hydro Pneumatic Energy Storage for Offshore Green Hydrogen Generation - HydroGenEration, Grant Agreement Ref.: EWA 64/22", is financed by the Energy and Water Agency under the National Strategy for Research and Innovation in Energy and Water (2021-2030).
[1] The project “Wind-driven Offshore Hydrogen Production with Electricity and Flow Stabilisation (WIND4H2)” was supported through the Maritime Seed Award (MarSA) 2019; a joint initiative between Transport Malta (formerly Malta Marittima) and the University of Malta, supported by the TAKEOFF Business Incubator, within the Knowledge Transfer Office, and the Centre for Entrepreneurship and Business Incubation (CEBI) at the University of Malta.
[2] The Light Detection and Ranging (LiDAR) system was purchased through the European Regional Development Fund for the setting up of a Solar Laboratory (ERDF 335), part-financed by the European Union.
[3] The help and support of Din L-Art Ħelwa – National Trust of Malta, for hosting the LiDAR wind measurement equipment at the Qalet Marku Tower, Baħar iċ-Ċagħaq, Malta, is hereby acknowledged.